If you've been around motorized vehicles for any length
of time, you have probably been exposed to the great torque
vs. power debate at some point. If not, it goes like this:
"Torque
is what makes a bike accelerate, not power."
Wrong.
Torque and power
are inescapably linked by the fact that horsepower equals
torque (in ft-pounds) times RPM divided by 5250, so people
who talk as if they are independent are not portraying the
full picture. If you have a given torque curve for an engine,
you have the horsepower curve also. Knowing how these two
numbers work with each other will help you understand what
you might read.
First, as usual,
a few definitions.
Torque
is a twisting force applied to an object, like a wheel or
a crankshaft. Note that motion is not required for torque
to exist! If you stand on a lug wrench that is on a frozen
lug bolt, you are applying a torque to that bolt even though
there may be no movement. For our purposes, we will consider
that torque is measured in pounds-force feet (lbf-ft) meaning
the equivalent of a given force, in pounds, acting on the
end of a lever of length in feet. For example, standing
with 180 pounds body weight on a lug wrench one foot long
yields 180 lbf-ft of torque. A child of 90 pounds standing
on a two-foot lug wrench applies the same torque.
Work
is the application of force over a distance. Unfortunately,
the units used are the same (pounds times feet) but we write
this as ft-lb just to distinguish it. The real difference
is that in this case, the "feet" part means feet
of movement. If you push on a car with 100 pounds of force
and maintain that for 30 feet, you have done 3000 ft-lb
of work. An easier example is lifting a weight (in pounds)
a given distance (in feet). If you use some sort of mechanical
advantage, like a winch, you will do the same amount of
work because by halving the effort required, you will have
to double the distance through which you apply the force
to achive the same objective.
Power
is the application of work within a finite time. 550 ft-lb
of work in one second is one horsepower.
So, let's first
go through the numbers to get from torque to horsepower.
Pushing with 87.5 pounds (force) on the end of our 1-foot
lug wrench applies a torque of 87.5 lbf-ft. No motion yet,
so no work and no power. But now let's say the lug bolt
loosens slightly and starts to turn, but that same 87.5
pounds of force is needed to keep the wrench turning. For
every revolution of the wrench, you are applying 87.5 pounds
of force over a distance of (2 * pi * 1 foot) or 6.28 feet,
the circumference of the circle that your hand is making,
for a total of 550 ft-lb of work. It's only when this system
is actually moving that work is being performed. From here,
it's a quick step to say that if you work fast enough to
turn that wrench once per second, then you are doing 550
ft-lb of work per second, which means you are applying one
horsepower.
By the definitions
we can see that HP is directly proportional to torque and
RPM. "Directly proportional" means there may be
a multiplyer involved, so let's find it using our example
numbers, remembering that 1 revolution per second is 60
RPM:
torque * RPM
* constant = hp
87.5 lbf-ft
* 60 rev/min * X = 1 hp
X = 1 / (60
* 87.5) = 1/5250
torque * RPM
* 1/5250 = hp
hp = (torque
* RPM) / 5250
For internal
combustion engines, torque is always given at a certain
RPM because they can't generate any torque when they aren't
moving. Once they are running fast enough to sustain their
own operation, the force that they are exerting against
a load can be measured, and the speed at which they are
turning can be measured, so the torque (and therefore power)
numbers become known.
So, if there
is such a fixed relationship between torque and power, why
do some people say that a certain engine has lots of power,
but no torque? Remember that the connection between torque
and power is rotational speed. A sportbike motor might generate
150hp at 14,000 RPM but the torque at that RPM is very small;
about 53 ft-lbs. In comparison, a large-displacement twin
might peak at 100 hp at 7000 RPM. The torque applied at
the twin's 7000 rpm, 75 ft-lbs, is greater than the torque
applied at the sport bike's 14,000 rpm but the sport bike
makes up for it with a lot more engine speed and ends up
with more horsepower.
The street, though,
complicates things because the sport bike will probably
not be ridden at 14,000 RPM. At 5000 RPM, the twin would
likely have more power. This is an artificial handicap;
the sport bike wasn't meant to be ridden at that speed since
it generates its power by sending the RPM part of the equation
sky-high. For street riding, the twin is easier to ride,
less prone to stalling as you pull away from a light, and
you get that satisfying "oomph" when you twist
the throttle. But as the RPM increases, the twin runs out
of breath and the race bike, although the torque is low
and probably getting lower, continues to make more and more
power until it hits its peak above 10,000 rpm.
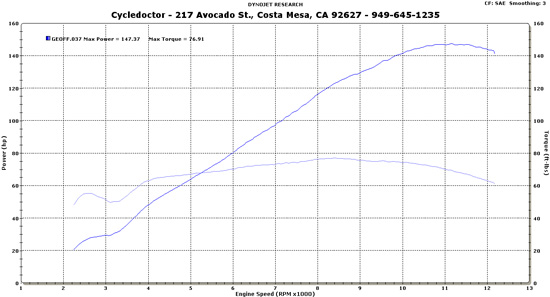
Engines are designed
for their intended use. Our twins are designed to yield
fairly high torque values at low RPM, because this makes
them easy to ride in day-to-day life, and Harley-Davidsons
have their torque concentrated even lower in the RPM range
than BMWs do. Low-end torque is accomplished by several
design traits, one being small valves and intake tubes which
create high air velocity into the cylinder for good fuel
mix at low speed.
Those effects
tend to become a restriction at high RPM, which means that
engines intended for high RPM end up with larger valves,
larger air intakes, smaller cylinders and other things that
let them continue to breathe when other engines start to
gasp. Race bike engines have fairly small displacement,
which limits the torque that can be produced at the crank.
They apply that torque at much higher speeds to get high
horsepower (and who can argue that those bikes don't accelerate
quickly?).
To a lesser extent,
BMW varies these techniques for different bikes. The GS
series has narrower intake tubes to give a faster intake
charge, giving better fuel/air mixing and better torque
at low RPM. Since this becomes a bottleneck at higher RPM,
the "power" engine in the RS and RT bikes have
larger intake tubes. Swapping the GS tubes into an RS or
RT is a common retrofit, as it makes the bike torquier at
low RPM where most of us ride. Newer technology in cars,
like variable valve timing and variable intake tract length,
can give motors the best of both worlds by increasing torque
at higher RPM without giving it up at low RPM. Incidentally,
Honda has variable valve timing on a motorcycle now.
But to get back
to the main point, it is power that moves our bikes down
the road. Yes, torque provides the pushing force through
the drivetrain, but it needs to happen at some given speed,
and those two factors define "power."
Why does torque
drop after a certain RPM?
Torque starts
to decrease because the engine cannot breathe as well. Due
to the speed, the cylinder does not fill with air as well.
A designer can get around this problem with "tuned
intake" which sets up a resonance to pack the cylinder
with air, but it only happens at a certain RPM. The next
evolution of design is to make a variable system which packs
the cylinders with air at all RPM; this is usually called
"variable tuned intake runners" or something like
that and involves valves which open and close to create
a different size for the airbox and manifold.
Why does power
continue to increase after torque decreases?
Remember that
the power is essentially the product of the RPM and the
torque. At first, decrease in torque is small and is not
enough to offset the increasing RPM, so the overall product
still increases. Eventually the decrease in torque becomes
large enough that it outweighs the increase in RPM and we
see the power start to drop. Because of this, the power
peak will always be after the torque peak.
-Author unknown
Article 2